Introduction
Probiotics are live, non-pathogenic microorganisms consumed by target species, which may be humans, animals, or avian species (Huys et al., 2013). Among probiotics, lactic acid bacteria (LAB) are of particular interest to the food industry, since these bacteria have generally regarded as safe (GRAS) status (Altuntas, 2013). Through clinical, animal, and cellular experiments, researchers have shown that probiotic preparations consisting of dead cells and their metabolites can also be biological response modifiers (Taverniti and Guglielmetti, 2011). This paradox of probiotics has been concisely reviewed by Adams (2010) and Taverniti and Guglielmetti (2011), who reported probiotics as paraprobiotics or ghost probiotics. The impact of probiotics can be attributed to their molecular components, and their effects can be mediated by either live or dead cells. Nevertheless, scientific evidence implies that inactivated microbes and their metabolites positively affect human and animal health (Adams 2010; Hor and Liong, 2014; Kataria et al., 2009). Accordingly, products designed with heat-killed strain Lactobacillus acidophilus (L. acidophilus) from human origin Lactéol strain (Coconnier et al., 1997) have been imported and marketed in China since early 1998 (PUMC Pharmaceutical Co., Ltd). Thus, the therapeutic effects of genus Lactobaccillus are well known, and their actions against infections and bacterial pathogens in different types of food products are in high demand as well (Altuntas, 2013).
Currently, a few cultures have already been introduced to the market as starters or bio-protective cultures with the purpose of ensuring microbiological safety (Aymerich et al., 2008). For example, a mixed culture of Pediococcus acidilactici and Lactobacillus curvatus in Bactoferm F-Lc has been patented by Christian Hansen as an antilisterial culture in fermented sausages (Aymerich et al., 2008). Those cultures produce pediocin and sakacin A, respectively. Lactobacillus sakei B-2 and Leuconostoc carnosum (Lc. carnosum) 4010 (B-SF-43) are also used as bio-protective cultures for vacuum-packed and meat products (Aymerich et al., 2008). Pathogenic spoilage bacteria such as Listeria monocytogenes, Salmonella species, Escherichia coli O157:H7, Staphylococcus aureus, Leuconostoc mesenteroides, Lc. carnosum, Brochothrix thermosphacta, and other pathogens were shown to be isolated from ready-to-eat meat products and suppressed using LAB (Aymerich et al., 2008). A couple of reports have shown that Bifidobacterium breve, L. acidophilus, and Lactobacillus casei (L. casei) reduce viability of the infection pathogen Cronobacter sakazakii (C. sakazakii), formerly Enterobacter sakazakii, which is present in commercially produced infant formula (Iversen and Forsythe, 2004).
Cronobacter species have been detected as causative agents of infection in both adults and infants. It was reported that contamination levels of C. sakazakii are usually <1.0 colony forming unit (CFU)/100 g in powdered infant formula, as determined by the most probable number method (Kornacki, 2014). Contamination incidence in international surveys has been shown to range from 2.4 to 14% (Iversen and Forsythe, 2004; Muytjens et al., 1988), whereas United States Food and Drug Administration field surveys reported 6.6% incidence (Zink, 2003). The presence of Cronobacter in manufacturing plants is presumably due to post-processing, e.g., during dry-mixing of the finished product, filling, and packaging (Muytjens et al., 1988). It has been reported that control of Cronobacter species is very difficult in a processing environment due to the widespread nature of the organism. Common ingredients such as lactose, whey and soy protein concentrates, vegetable oil, vitamin and minerals, corn syrup, and corn maltodextrin are consumed as potential nutrients of Cronobacter species (Kornacki, 2014). Scientific investigation of the physiology of this pathogenic bacterium has confirmed the biofilm-forming ability and high resistance of Cronobacter species against antibacterial agents. There have been many reports on inactivation of Cronobacter species using trans-cinnamaldehyde (Amalaradjou and Venkitanarayanan, 2011), lactic acid, copper (II), monolaurin, and antimicrobial peptides such as caseicin A and caseicin B from L. acidophilus DPC 6026 (Amalaradjou and Venkitanarayanan, 2011; Hayes et al., 2009). However, these natural compounds are not applicable for application in infant milk formula due to their strong flavors. Even though propionic acid and acetic acid have GRAS status, they are currently not included in Codex standards for infant formula (Kent et al., 2015).
The purpose of this research was to identify natural and safe bio-preservatives that are industrially operable and safe for infants. Fermented milk with L. acidophilus n.v. Er2 317/402 strain Narine is a supplementary source of nutrition for newborns and used as an alternative to baby formula under the trade name Narine. Narine baby food has a time proven track record as a safe baby nutrition formulation (Mkrtchyan et al., 2010). L. acidophilus n.v. Er2 317/402 strain Narine is known as a health beneficial functional probiotic culture. The strain Narine suppresses pathogenic microorganisms, as classified by the World Health Organization (Mkrtchyan et al., 2010). Therefore, this study was performed to assess if Narine could be employed as a food preservative by inhibiting growth of C. sakazakii.
Materials and Methods
Lyophilized L. acidophilus n.v. Er2 317/402 strain Narine (Narine-L) originally isolated from feces of newborn babies was purchased from Atlas R&D Inc. (Cambridge, Canada) in encapsulated form. To measure the viability of Narine cells, 100 mg of Narine-L was suspended in 50 mL of sterilized skim milk. After mixing very gently, the suspension was kept in a refrigerator at 4℃ for 24 h to revive bacterial cells. The suspension was mixed periodically with specific time intervals of 8-10 h. After 24 h, the entire 50 mL suspension was sent to Lab Services Division (Guelph, Canada) for colony counting. Enumeration of L. acidophilus n.v. Er2 317/402 strain Narine was carried out on an automated TEMPO system (bioMérieux, France).
C. sakazakii ATCC 29544 was purchased from the American Type Culture Collection (Rockville, USA) and used as an artificial contaminant microorganism. C. sakazakii was cultivated overnight in brain heart infusion (BHI) broth and on violet red bile glucose (VRBG) agar plates (MB Cell, USA). Yeast extract, peptone, L-cysteine, Tween-80, and skim milk were purchased from (Difco, USA). Glucose, phosphotungstic acid, and tetracycline were purchased from Sigma (USA).
To determine whether or not the antimicrobial activity of L. acidophilus n.v. Er2 317/402 strain Narine is due to its metabolites, cells were subjected to heat-kill treatment. Narine-L cells were heat-killed at 80℃ for 30 min and named Narine-heat treated (Narine-HT). Further, extracellular extracts were collected from Narine-HT by sequential extraction using water, sonication, and centrifugation; Narine-HT (2 g) was hydrated with 10 mL of sterile water, vortexed, and crushed in a Restch mill (Haan, Germany) for 1 min. Supernatant was obtained by high speed centrifugation at 11,000 g for 15 min at 4℃, followed by filtration through a 0.25 μm microfilter (Advantec, Japan) to remove bacterial cells. The resulting filtrates were lyophilized and designated as Narine crude cell-free extract (Narine-CCFE) for further evaluation.
The agar well diffusion method was used to measure the antimicrobial activities of Narine-L, Narine-HT, and Narine-CCFE against C. sakazakii. In brief, overnight culture of C. sakazakii (1 mL) was mixed with 100 mL of semi-solid BHI medium containing 0.7% agar, poured as a second layer, and allowed to solidify before cutting wells as previously described by Awaisheh et al. (2013). Each 30 mg of Narine-L, Narine-HT, and Narine-CCFE was applied to 5 mm wells and then incubated at 30℃ overnight. Inhibition zone around each well was measured and expressed in units (0.1 mm = 1 U). The diameter of the halo was deducted from the diameter of the well (15 mm = 150 U). Tetracycline (0.01 μg/mL) was used as a positive control. The test was performed in triplicate and repeated twice.
Ten grams of commercial infant powdered milk (Maeil Dairy Industry Co., Korea) was mixed with 90 mL of sterile distilled water under aseptic conditions to make reconstituted powdered infant formula (RPIF). Then, 100 μL of C. sakazakii (108 CFU/mL) was subjected to 10 mL of RPIF with 1 g each of Narine-L and Narine-HT. A negative control was prepared without both Narine-L and Narine-HT. In a subsequent experiment, samples (1 mL) were aseptically collected every 0, 3, and 6 h of incubation at 37℃ and serially diluted in 0.1% peptone water for enumeration of C. sakazakii. From the appropriate dilution, 100 μL of each sample was spread onto the surface of a VRBG agar plate to determine the number of viable C. sakazakii.
To observe the morphology of C. sakazakii cells after treatment with Narine-CCFE, C. sakazakii cells were subjected to TEM according to the method recommended by Coconnier et al. (1997). After negative staining with 2% phosphotungstic acid (w/v), cell morphology was examined using TEM (H-7600; Hitachi, Japan) at 80 kV.
Results and Discussion
The antimicrobial activity of Narine against C. sakazakii (108 CFU/mL) was expressed as the diameter (U) of the inhibition zone around Narine-L, Narine-HT, and Narine-CCFE. The zone ranged from 150 to 200 U (Fig. 1). The same amounts (30 mg) of Narine-L, Narine-HT, and Narine-CCFE were tested against C. sakazakii, and the highest inhibitory activities (200 U) were observed with Narine-CCFE, which is a soluble caramelized material. The component(s) in Narine-CCFE providing antimicrobial activity was acidic or required an acidic environment to ideally develop antimicrobial activities. Treated Narine-CCFE with phosphate buffer at neutral pH did not exhibit any antimicrobial activity (data not shown). In recent studies, it was demonstrated that a mixture of caprylic acid, citric acid, and vanillin had a destructive effect on a number of Cronobacter strains (Choi et al., 2013). The authors specified that there was significant synergistic activity of the antimicrobials against the pathogens (Choi et al., 2013). In this respect, it seems that the acid components in Narine-CCFE are potent antimicrobials, possibly due to the greater proportion of acid molecules in undissociated form, which rapidly enter Cronobacter cells and increase levels of cytoplasmic acidification in bacteria.
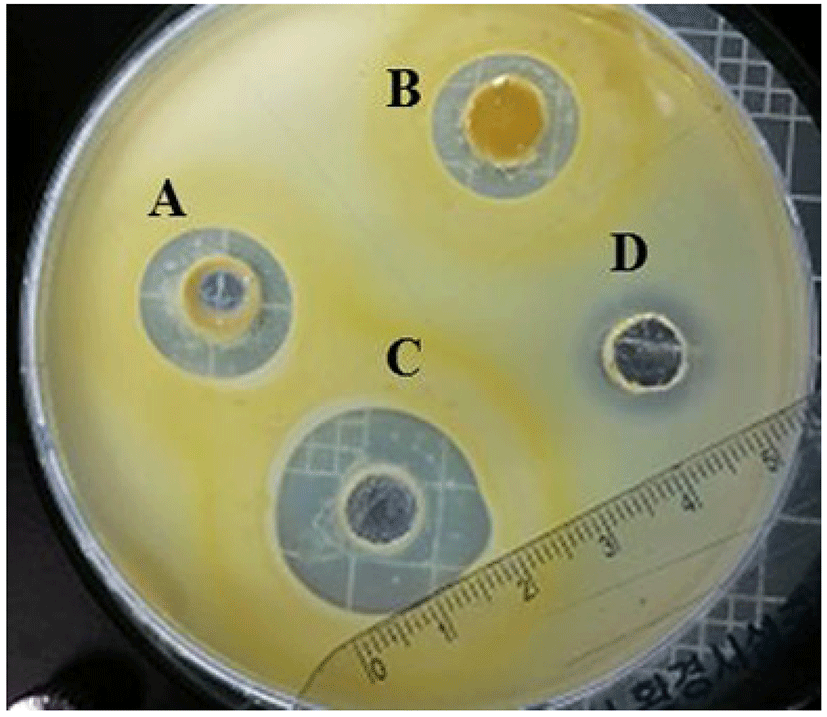
Furthermore, Narine-L and Narine-HT were used in an infant formula model to reduce 1.62×108-5.36×109 CFU/mL after 3 and 6 h (Table 1). The result of enumeration for C. sakazakii were counted on VRBG agar plate before and after treatment with Narine-L and Narine-HT, respectively. After treatment with Narine-L and Narine-HT for 3 h and 6 h at 37℃, less than ≤ 107 CFU/mL of C. sakazakii were detected in RPIF. On the other hand, without treatment of Narine-L and Narine-HT, the population of C. sakazakii increased up to 5.36×109 after 6 h.
Sample | 0 h | 3 h | 6 h |
---|---|---|---|
Control a) | 1.62×108 | 3.17×109 | 5.36×109 |
Narine-lyophilizedb) | 1.62×108 | ≤107 | ≤107 |
Narine-heat treated c) | 1.62×108 | ≤107 | ≤107 |
a)Reconstituted powdered infant formula artificially contaminated with Cronobacter sakazakii. b)Reconstituted powdered infant formula artificially contaminated with Cronobacter sakazakii under treatment with Narine-lyophilized. c)Reconstituted powdered infant formula artificially contaminated with Cronobacter sakazakii under treatment with Narine-heat treated.
To further characterize the antimicrobial effect of Narine-CCFE, we used TEM (Eltson and Zuber, 2006) to examine C. sakazakii cells before and after treatment with Narine-CCFE (Fig. 2). Zoomed micrograph shows live cells of C. sakazakii, which are transparent (Fig. 2A), whereas after less than 3 h of treatment with 30 mg of Narine-CCFE, there are detectable examples of altered cell membrane morphology (Fig. 2B). A similar observation was made for the spent culture supernatant of human L. acidophilus strain LB (LB-spent culture supernatant), producing antimicrobial activity against a wide range of gram-negative and gram-positive pathogens (Canducci et al., 2000; Coconnieret et al., 1997). The TEM images corroborate the previous studies performed by Coconnieret et al. (1997), where TEM images showed a damaged cell membrane morphology. Close examination of both images, Salmonella Typhimurium SL 1344 treated with LB-spent culture supernatant and our C. sakazakii treated with Narine-CCFE, showed the same altered cell envelope. Feliciano et al. (2012) reported that inactivated bacterial cells showed a less defined envelope along with a rugged cell wall, suggesting that inactivated cells appeared due to reduced integrity of the cell envelope and aggregation of cytoplasmic components.
In a previous work, L. acidophilus n.v. Er2 317/402 strain Narine, grown on chemically-defined synthetic medium, was reported to have pathogen-killing effects due to acidocin LCHV (Mkrtchyan et al., 2010). However, we were unable to detect the same acidocin LCHV when Narine was grown in industrially available skim milk (data not shown). Nevertheless, we provide evidence that this inhibition occurred when the pH of Narine-CCFE was acidic while no growth inhibition occurred when the pH of Narine-CCFE was neutral. Our findings are in agreement with those reported by Servin (2004), who showed that antimicrobial activity resulted from an acidic pH, which functions as a permeabilizer of the outer membrane of gram-negative bacteria and may potentiate the effects of other antimicrobial substances.
A few studies investigated the direct inhibitory effect of Lactobacillus on Cronobacter. One study demonstrated the beneficial effects of human strains of L. casei and L. acidophilus against C. sakazakii (Awaisheh et al., 2013). Initially, LAB strains were isolated from infant stool samples and screened to show bacteriocin production by using cell-free supernatant against C. sakazakii in RPIF. The results showed that L. casei and L. acidophilus reduced C. sakazakii (Awaisheh et al., 2013). In a present work, human strain L. acidophilus n.v. Er2 317/402 Narine and its metabolites inhibited C. sakazakii. With regard to Cronobacter, Kim et al. (2015) found that strains Lactobacillus kefiri and Candida kefyr either individually or in combination with their supernatants significantly reduced growth of Cronobacter strains in RPIF. However, it is known that kefir grains are a combination of LAB, acetic acid bacteria, and yeast, whereas L. acidophilus n.v. Er2 317/402 Narine is a pure culture isolated from newborn baby feces and is already used in baby food.
Conclusions
This preliminary study demonstrated the antimicrobial activity of L. acidophilus n.v. Er2 317/402 strain Narine against C. sakazakii in vitro. Narine-L, Narine-HT, and Narine-CCFE of 30 mg showed inhibition zones from 150 to 200 U, with the largest inhibition (200 U) induced by Narine-CCFE. One gram each of Narine-L and Narine-HT was added to 10 mL of artificially contaminated RPIF containing 100 μL of C. sakazakii (1.62×108 CFU/mL), and the results showed that Narine-L and Narine-HT inhibited 1.62×108-5.36×109 CFU/mL of C. sakazakii throughout 6 h at 37℃. TEM analysis confirmed the susceptibility of C. sakazakii by L. acidophilus n.v. Er2 317/402 Narine. The administration of Narine strains in infant milk formula might promote safety of infant foods. Further studies are needed to investigate the metabolite components responsible for antimicrobial activity.