Introduction
Several projections have suggested that world population will reach over 9 billion by 2050 (Grafton et al., 2015; Park and Yun, 2018). This increase in population requires approximately double the current food production (Belluco et al., 2013). Alarmingly, global warming is gradually decreasing the areas used for food production worldwide (Dobermann et al., 2017). The climate change and the environmental destruction from industrial development also negatively affect the food productivity (van Huis and Oonincx, 2017). In light of worsening resource shortage, several foods have been proposed as alternatives, with insects receiving the most attention (Patel et al., 2019). Insects are institutionally accepted as a food in many regions and historically consumed (Murefu et al., 2019), providing sufficient nutritional value for humans (Zielinska et al., 2018). However, the rapid increase in food production through technological advancement has largely eliminated insects from our diets (Gao et al., 2018).
The reappearance of insects as a viable food group can be attributed to their nutritional, environmental, and economic value (Nongonierma and FitzGerald, 2017). The increased scrutiny of edible insects is part of a multifaceted strategies for achieving global food security (van Huis, 2015). In general, insects have high protein content and excellent production efficiency compared with other conventional food groups (Kohler et al., 2019; Nongonierma and FitzGerald, 2017; Zielinska et al., 2015). This characteristic is particularly valuable given that future protein consumption is expected to increase, but food supply declines (Gao et al., 2018; Kohler et al., 2019; Patel et al., 2019). Currently, Europe and the United States have the fastest growing edible-insect industry, where a trend is associated with high meat intake (Mlcek et al., 2014). Moreover, it is recognized that a steady increase in the global market size of the insect industry, with applications reaching beyond food into material and drug development (van Thielen et al., 2019).
Despite the numerous practical advantages, many barriers remain in insect-food development because the concept of insect foods is not similar with conventional Western eating habit (Murefu et al., 2019). Currently, insect foods are in a transitional stage, and a promising new technique involves developing new food products via combining protein-processing technology with insects (Kohler et al., 2019; Patel et al., 2019). This review aims to provide a much needed overview of current research on insect-food development. As we will demonstrate, the insect industry is on track to become a successful protein resource that will lead the global market.
Industrial Applications of Insects as Food and Feed Sources
For hundreds of years, native cultures in Asia, South America, Africa, and Europe include the consumption of various species of insects (Dobermann et al., 2017; Raheem et al., 2018). Approximately 2,000 insect species are consumed in at least 113 countries (Yen, 2009). A survey of markets in Bangkok, Thailand, for instance, identified 164 insect species being sold for food (Yhoung-aree, 2010). The most commonly eaten insects are beetles, caterpillars, bees, ants, crickets, grasshoppers, and locusts (Raheem et al., 2018). In Zimbabwe, Zambia, and Nigeria, edible insects are commonly available in school cafeterias and open markets, forming a profitable business (Mutungi et al., 2019). Indeed, up to 50% of dietary protein is derived from insects, and they actually have higher market value than other protein sources (Dobermann et al., 2017). Some insects are appreciated for their organoleptic characteristics and consumed in high-class restaurants (DeFoliart, 1999). For example, escamoles (ant eggs) are considered a delicate gourmet dish in Mexico, Laos, Cambodia, and Europe (Ramos‐Elorduy, 1997).
An interest in edible insects has increased rapidly because the Food and Agriculture Organization (FAO) has begun promoting insects as viable dietary options for humans (van Huis et al., 2013). Globally, the edible-insect market is expected to exceed USD 522 million by 2023 (Han et al., 2017). Since 2012, the Korean edible-insect market (especially for human consumption) has made major progress with government support and successful research endeavors.
However, lingering negative perceptions of insects hamper global market expansion and limit insects as a mainstream dining option, which may be related to the fact that people are skeptical to novel foods due to general neophobic tendencies (Dobermann et al., 2017). Thus, inexperienced consumers perceive insects as a source of fear or disgust, have strong rejection of insects as a normal food in their diet, and totally neglect their high nutritive value (van Huis, 2016). Overcoming such intrinsic attitudes are one of the main challenges facing the edible-insect industry (Yen, 2009). Fortunately, the positive social perception of novel foods does lead to its consumption (Yen, 2010), as consumer attitudes are driven multiple factors (e.g., food-choice motives) beyond neophobia (van Thielen et al., 2019). Thus, initiatives promoting edible insects should emphasize their practical value, which can create consumer demand (Sun-Waterhouse et al., 2016). Governments and NGOs can provide the information about benefits related to nutrition and environmental sustainability (Ruby et al., 2015). Tasting events or educational workshops can also provide opportunities to learn about edible insects (Han et al., 2017). Another method for improving consumer perception is the creation of cookbooks with insect recipes (Raheem et al., 2018). Overall, the repeated positive exposure to edible insects raises awareness and could encourage consumption (van Thielen et al., 2019).
Moreover, an increase in accessibility to edible insects is to develop insect-based ingredients instead of final food products showing their original appearance (Mishyna et al., 2019; Nongonierma and FitzGerald, 2017; Purschke et al., 2018; Yi et al., 2016). Incorporating edible insects in already-familiar foods may be more acceptable for an insect-phobic culture than providing insects directly as a food option, and using insects as food ingredients is beneficial for the formation of sustainable business models (Han et al., 2017).
A major hurdle in the edible insect industry is the lack of systematic work to guarantee safety and shelf-life (van Huis, 2016). Insect farming also requires standardization and quality control, a goal that requires government legislation and regulations (Han et al., 2017).
High nutritional value, minimal space requirements, and low environmental impact combine to make insects an appealing option for animal feed. Another major advantage is that insects are already used for the natural part of many animal diets (Rumpold and Schlüter, 2013b; Veldkamp and Bosch, 2015). Insect-based animal feeds are particularly attractive when considering the cost of standard feeds, currently accounting for 70% of livestock-production expenses (van Huis et al., 2013).
The most promising, well-studied candidates for industrial feed production are black soldier flies, larvae, yellow mealworms, silkworms, grasshoppers, and termites (Dobermann et al., 2017). Such previous research has revealed that insect meal can partially replace commercial meal in broiler feed, particularly protein sources. For example, housefly-larvae meal can replace 4% of the fish meal in broiler diets without negative effects on carcass weight and feed efficiency (Awoniyi et al., 2003). Another report indicated that broiler diets containing 31%, 26%, and 20% soybean meal can be successfully modified to contain 0%, 5%, and 10% dried yellow mealworm, respectively (Ramos-Elorduy et al., 2002). Furthermore, growth performance and carcass quality could be improved when broiler chicks were fed a diet containing 10%–15% housefly larvae (Hwangbo et al., 2009). As compared with commercial corn/soy-based diets, a housefly-larvae-based diet significantly increased the carcass weight, total feed intake, and average daily gain of broiler chickens (Pretorius, 2011). However, a more recent study found that replacing soybean oil with black-soldier-fly-larvae meal has no impacts on the growth performance of broilers (Schiavone et al., 2017). In addition to the nutritional value, insect-based feed could have a further advantage in improving the taste of final meat products. In the Philippines, for example, consumers prefer the taste of pasture-grown chickens fed with grasshoppers, resulting in higher price compared with chickens on commercial feed (Litton, 1993).
Insect-based feeds have also been tested in egg-producing poultry. The replacing fish meal with dried mealworm increased egg production by 2.4% (Wang et al., 1996). Fully replacing the protein content with larvae meal in a laying-hen diet did not negative affect feed intake, feed conversion efficiency, egg production hen health, and immune status (Marono et al., 2017).
Smallholder farms in Asia and Africa commonly use insects as fish feed (Dobermann et al., 2017). Housefly-larvae meal can replace up to 75% of fishmeal in Nile tilapia diets without any adverse effects (Wang et al., 2017). Replacing fish meal with black-soldier-fly meal in diets does not alter the odor, flavor, or texture of Atlantic salmon (Salmo salar) (Lock et al., 2016). Likewise, mealworms can successfully replace 40%–80% of the standard catfish (Ameiurus melas Raf.) diet without adverse effects on growth performance (Roncarati et al., 2015). Another viable alternative to fish meal is silkworm pupa, which was tested successfully for African catfish (Clarias gariepinus) fingerling diets (Kurbanov et al., 2015). In carp (Cyprinus carpio), a silkworm-meal diet was superior to a leaf-meal diet for improving nutrient digestibility, nutrient retention, and feed conversion efficiency (Swamy and Devaraj, 1994).
In summary, existing studies clearly demonstrate that insects are a promising protein source for animal feeds and has the potential to meet increasing global demand (Rumpold and Schlüter, 2013b). Before the mass-production of such insect-based feeds, however, governments and companies should aim to address health and safety concerns related to edible insects, such as the presence of anti-nutrient properties (Dobermann et al., 2017).
Value of Edible Insects as Food Resources
Insect nutritional value varies with diet, developmental stage, sex, species, growth environment, and measurement methods (van Huis and Oonincx, 2017). Nevertheless, researchers generally agree that insects are extremely rich in protein, fat, and vitamins (Rumpold and Schluter, 2013a). Nutrient compositions of edible insects as published in literatures (based on dry matter) is summarized in Table 1. Nitrogen is a critical nutrient, and proteins directly involved in N supply comprise 16.5% of an adult human body (Melo et al., 2011). On average, the protein content of edible insects ranges 35%–60% dry weight or 10%–25% fresh weight (Melo et al., 2011; Schluter et al., 2017), which are higher than plant protein sources, including cereal, soybeans, and lentils (Bukkens, 1997). At the upper range, insects provide more protein than even meat and chicken eggs (Mlcek et al., 2014). Edible insects in Orthoptera (crickets, grasshoppers, locusts) are particularly protein-rich (Rumpold and Schlüter, 2013a). However, insect protein digestibility is highly variable due to the presence of a hard exoskeleton (van Huis, 2016). Exoskeletons with high proportion of chitin component are especially difficult to digest (Schluter et al., 2017). Indeed, we currently do not know whether humans are capable of digesting chitin (Muzzarelli et al., 2012). Of course, the removal of exoskeleton through a part of processing is a viable option (Rumpold and Schlüter, 2013b). Some studies have found that insect protein digestibility is 77%–98% without after the exoskeleton (Defoliart, 1992).
Type of insects | Scientific name | Protein content (%) | Fat content (%) | Carbohydrates content (%) | Reference |
---|---|---|---|---|---|
Larvae | Allomyrina dichotoma | 54.18 | 20.24 | -1) | Ghosh et al. (2017) |
Anaphe infracta | 20.00 | 15.20 | - | Banjo et al. (2006) | |
Anaphe recticulata | 23.00 | 10.20 | - | Banjo et al. (2006) | |
Anaphe venata | 25.70 | 23.21 | - | Banjo et al. (2006) | |
Gonimbrasia belina | 56.95 | 10.00 | 7.80 | Siulapwa et al. (2012) | |
Gynanisa maja | 55.92 | 12.10 | 10.70 | Siulapwa et al. (2012) | |
Protaetia brevitarsis | 44.23 | 15.36 | - | Ghosh et al. (2017) | |
Rhynchophorus phoenicis | 22.06 | 66.61 | 5.53 | Ekpo and Onigbinde (2005) | |
Tenebrio molitor | 46.44 | 32.70 | - | Ravzanaadii et al. (2012) | |
Beetle | Heteroligus meles | 38.10 | 32.01 | 20.10 | Jonathan (2012) |
Oryctes boas | 26.00 | 1.50 | - | Banjo et al. (2006) | |
Rhynchophorus phoenicis | 50.01 | 21.12 | 20.23 | Jonathan (2012) | |
Rhynchophorus phoenicis | 28.42 | 31.40 | - | Banjo et al. (2006) | |
Grasshopper | Ruspolia differens | 44.59 | 49.00 | 8.40 | Siulapwa et al. (2012) |
Zonocerus variegatus | 26.80 | 3.80 | - | Banjo et al. (2006) | |
Cricket | Brachytrypes spp. | 6.25 | 2.34 | - | Banjo et al. (2006) |
Gryllus bimaculatus | 58.32 | 11.88 | - | Ghosh et al. (2017) | |
Teleogryllus emma | 55.65 | 25.14 | - | Ghosh et al. (2017) | |
Termites | Macrotermes bellicosus | 20.10 | 28.20 | - | Banjo et al. (2006) |
Macrotermes falciger | 43.26 | 43.00 | 32.80 | Siulapwa et al. (2012) | |
Macrotermes notalensis | 22.10 | 22.50 | - | Banjo et al. (2006) | |
Bee | Apis mellifera | 21.00 | 12.30 | - | Banjo et al. (2006) |
Dragonfly | Aeschna multicolor | 54.24 | 16.72 | - | Ramos-Elorduy et al. (1998) |
Anax sp. | 26.22 | 22.93 | - | Ramos-Elorduy et al. (1998) |
The second largest component of insect nutrient composition is fat (Mlcek et al., 2014). Various factors such as species, sex, reproduction stage, season, diet, and habitat all combine to influence insect fat content (Schluter et al., 2017). Orthoptera, Lepidoptera (caterpillars), cockroaches (blattodea), Isoptera (termites), Hemiptera, and Coleoptera (beetles, grubs) have the averaged fat content of 13.41%, 27.66%, 29.90%, 32.74%, 30.26%, and 33.40%, respectively (Rumpold and Schluter, 2013a). Larvae and pupae have more fat than adult insect (Mlcek et al., 2014). In addition, females are fatty than males (de Castro et al., 2018; Mlcek et al., 2014). The fatty acid profiles of insects are also dependent on species and diet (Schluter et al., 2017), although insects generally have more unsaturated fatty acids (UFA) compared to saturated fatty acids (SFA) (de Castro et al., 2018). On average, Hymenoptera (ants, bees, and wasps) and 41.97% for Isoptera (termites) have 30.83% and 41.97% SFA, respectively (Rumpold and Schluter, 2013a). A notable exception occurs in Imbrasia ertli catepillars, which possess SFA comprising 38% arachidic acid (C20:0) (Bukkens, 1997; Rumpold and Schluter, 2013a).
Carbohydrates in insects mainly exist in two forms of chitin and glycogen. The former is a polymer of N-acetyl-D-glucosamine that is the primary component of exoskeleton (Bukkens, 1997; van Huis et al., 2013), while the latter is an energy source stored in cells and muscle tissues (Schluter et al., 2017). The averaged carbohydrate content of edible insects ranges from 6.71% (stink bug) to 15.98% (cicada) (Mlcek et al., 2014).
Some insects (e.g., grasshoppers, crickets, termites, and mealworms) are rich in iron, zinc, calcium, copper, phosphorus, magnesium, and manganese. Invertebrates without a mineralized skeleton have very little calcium content (de Castro et al., 2018; Mlcek et al., 2014). Most edible insects have similar iron content to beef (Bukkens, 1997), but we currently know little about mineral bioavailability of insects (de Castro et al., 2018). A rare study found that consuming insects can provide the high proportions of daily mineral recommendations for humans, particularly in terms of iron (Latunde-Dada et al., 2016). The investigations of vitamin content are also insufficient, but available data indicate that edible insects contain carotene, vitamin B1, B2, B6, C, D, E, and K (Mlcek et al., 2014). In particular, Orthoptera and Coleoptera are rich in folic acid (Rumpold and Schlüter, 2013a).
As traditional animal-protein intake methods are called into question, insects are increasingly viewed as the food of the future (Sogari, 2015). In the Netherlands, the efforts to promote entomophagy include studies aimed at customizing insects for Western tastes, resulting in the sale of some insects (e.g., locusts, mealworms) at retail markets (Schösler et al., 2012). Insects have been processed into powder or meal to minimize visual associations and increase palatability (Bubler et al., 2016). In addition, researchers have been investigating the functional properties of insect proteins, including gelling capacity, foam capacity, emulsion capacity, and solubility in various buffers or solvents. In Table 2, previous studies regarding the food processing properties (functional properties) of edible insects are presented. Appropriate extraction methods (e.g., defatting, pH change, zymolysis, or sonication) are also being investigated (Mishyna et al., 2019; Nongonierma and FitzGerald, 2017; Purschke et al., 2018; Yi et al., 2016). These studies will help the use of edible insects as a food ingredient for producing traditional foods.
Type of insects | Scientific name | Observation | Reference |
---|---|---|---|
Larvae | Cirina forda | • Protein solubility | Omotoso (2006) |
• Oil absorption capacity | |||
• Foaming stability | |||
Larvae | Gryllodes sigillatus | • Protein solubility | Zielinska et al. (2018) |
Grasshopper | Schistocerca gregaria | • Water holding capacity | |
Cricket | Tenebrio molitor | • Oil holding capacity | |
• Foaming capacity | |||
• Emulsion capacity | |||
Larvae | Cirina forda | • Protein solubility | Osasona and Olaofe (2010) |
• Oil absorption capacity | |||
• Water absorption capacity | |||
• Foaming capacity | |||
• Emulsion capacity | |||
Larvae | Oryctes owariensis | • Oil absorption capacity | Assielou et al. (2015) |
Larvae | Bombyxmori L. | • Water absorption capacity | Omotoso (2015) |
• Foaming capacity | |||
• Foaming stability | |||
• Emulsion capacity | |||
• Emulsion stability | |||
Larvae | Tenebrio molitor | • Water binding capacity | Bubler et al. (2016) |
Larvae | Hermetia illucens | • Fat binding capacity | |
• Protein solubility | |||
Larvae | Tenebrio molitor | • Foaming capacity | Yi et al. (2013) |
Larvae | Zophobas morio | • Foaming stability | |
Larvae | Alphitobius diaperinus | • Gel formation | |
Cricket | Acheta domesticus | ||
Cockroach | Blaptica dubia |
Cultures that consume insects also tend to associate them with various health benefits beyond nutrition (Raheem et al., 2018). For example, caterpillar fungus supposedly has immunostimulatory and anti-cancer properties (Chen et al., 2009). In traditional Chinese medicine, male Antheraea pernyi is considered aphrodisiac (Chen et al., 2009), while ant-alcohol is also thought to enhance immunity and libido (Liu et al., 2004). Some evidence exists to suggest that termites (Macrotermes annandalei) may have immunostimulatory effects (Chen et al., 2009). Another insect historically considered to have beneficial health effects is the silkworm (Bombyx mori L.) (Kim et al., 2008). Recent analyses have identified a blood-glucose-lowering agent, resulting in the development of silkworm powder as a diabetic medicine in Korea, and such health benefit was also reported by the Chinese Ministry of Health and State Food and Drug Administration (Belluco et al., 2013; Nongonierma and FitzGerald, 2017).
The traditional claims of medicinal properties have resulted in multiple studies aiming to empirically determine the properties of edible insects. The several analyses of insect enzymatic hydrolysates have identified antioxidant and antidiabetic properties, as well as the ability to inhibit angiotensin converting enzyme (ACE) (de Castro et al., 2018; Nongonierma and FitzGerald, 2017). The hydrolysates of S. littoralis larvae exhibit 2,2′azino-bis(3-ethylbenzothiazoline-6-sulphonic acid) and 2,2-diphenyl-1-picrylhydrazyl scavenging activity that indicates a powerful antioxidant activity (Vercruysse et al., 2009).
In summary, the discovery of physiologically important substances contained in edible insects makes medicinal use a potentially important application (Han et al., 2017). However, given the current lack of scientific data, more researches are warranted to confirm the benefits of consuming insects as health foods or medicine.
Despite the obvious nutritional value of edible insect, the food safety issues of edible insects such as microorganism, allergic reaction, and toxicity may catch up with latent consumer (Rumpold and Schlüter, 2013a). Harmful factors affect food safety of edible insect is summarized in Table 3. In the perspective on microbial safety, insect gut is considered as a main habitat, and body surface and mouthparts are also included. Microbial colonization is reached vertically through ovary, egg capsule, spawning, and horizontally through feed and environment (Dematheis et al., 2012; Yun et al., 2014). Therefore, the use of insect as food sources may have a potential hazard as a pathogenic vector. In addition, the microbiota ratio in insect gut can be an important part being used as food sources, because the total amount of biomass from gut microbiota could account for 1%–10% of the body weight of insect (Douglas, 2015). In addition, it is practically difficult to remove gut part from edible insects (Douglas, 2015). Although insect-specific pathogenic microorganisms had no risk to human health, they can colonize only cells or tissues of insect. However, the contamination of human pathogenic microorganisms can occur, and this contamination may be regulated by controlled breeding condition with special care (Schluter et al., 2017). In allergic reactions, some edible insects had allergic cross-reactive proteins with arachnids and crustaceans, which are known as arthropods (Ribeiro et al., 2018). Furthermore, carmine used for food dye is obtained from the bodies of female cochineal insects and this dye could induce allergic reaction to some patients (Belluco et al., 2013). Considering that only a few studies regarding the allergic reaction of edible insects have been carried out, we need to pay attention to the unknown potential allergens contained in edible insects. Further study and investigation determining the hazard substances including allergens and toxic substances and their impacts on pathological symptom in human body should be performed for guaranteeing the safe use of edible insects as feed and food resources.
Historical and Current Consumption Patterns of Edible Insects
The lack of sophisticated tools and well-developed hunting ability mean that ancient cultures likely consumed insects frequently (Valadez, 2003). Upon the development of agriculture and domestication of livestock, however, insect-eating habits have been disappearing in the many regions (Abrams, 1987). The resultant abundance of food naturally could also cause a decrease in the need for insect consumption. With changing cultural mores, insects have evolved from a primary food source to snacks, luxury ingredients, and bait (Smeathman, 1781). However, some countries have still continued to use insects as ordinary food resources to this day. Below, we will introduce and discuss detailed examples of current patterns of insect consumption.
In Korea, canned silkworm pupae are sold in retail markets and processed as snacks. Additionally, grasshoppers (Oxya velox) are eaten in rural areas (Pemberton, 1994). In Japan, a dish called inago involves fried grasshoppers seasoned with soy sauce. Moreover, bee or wasp larvae/adults are considered expensive delicacies; they are eaten raw, boiled with soy sauce, or served with rice (DeFoliart, 1999). Entomophagy has existed in China for over 2000 years; approximately 324 species from 11 orders are consumed possibly (Feng et al., 2018). India has the developed multiple uses for insects, including silk, fertilizer, food, and medicine. Notably, eri silkworm (Samia ricini) pupae is a delicacy in northeastern India (Peigler, 1993). Overall, about 255 species are used as food, although the intensity varies depending on seasonal or regional differences in culture (Chakravorty, 2014). In Thailand, insects are an important source of protein, fat, and other nutrients; the villages of Ubon alone consume 20–60 g of insects daily (Sungpuag and Puwastien, 1983). Over 80 species are considered edible food resources (DeFoliart, 1999). Furthermore, Thailand’s Ministry of Public Health recommends insect consumption for rural communities to obtain necessary nutrients (DeFoliart, 1999), in addition, it has also spread from rural to urban markets (Yhoung-aree, 2010). The information on major edible insects consumed in Asia is summarized in Table 4.
Sago grub (Rhynchophorus ferrugineus) is a popular edible insect in Papua New Guinea and a main part of an annual grub festival (Mercer, 1993). In local regions, locusts, crickets, mole-crickets, mantises, and even spiders are consumed (Meyer‐Rochow and Changkija, 1997). Aboriginal tribes consumed a wide variety of insects from Cossidae, Noctuidae, Cerambycide, and bees (Macfarlane, 1978). In Australia, entomophagy is low among European-derived populations (Yen, 2010). However, the edible-insect market has increased dramatically alongside an interest in bushfood, and insects are now available as restaurant menus (Irvine, 1989). More information about the edible insects in Oceania are given in Table 5.
Edible insects play an important role in the food culture of Africa (Mutungi et al., 2019). Caterpillars and termites are the most popular insects, but the insects from other orders are also widely eaten (DeFoliart, 1999), including Lepidoptera, Orthoptera, and Coleoptera (Kelemu et al., 2015). In total, approximately 470 species are consumed, and eaten insects are summarized in Table 6. Some examples from specific countries include grasshoppers, considered a source of energy and protein in Uganda (Mbabazi, 2011). Additionally, edible insects could account for over 20% of the animal protein produced in the Democratic Congo, where more than 65 species are consumed (DeFoliart, 1999). Overall, entomophagy is widespread and important to human nutrition in Africa (Mutungi et al., 2019).
Major edible insects consumed in the Americas are shown in Table 7. Edible insects are a major protein source for Amazonian tribes, with women and children actively foraging for them (Dufour, 1987). In particular, Rhynchophorus palmarum and Atta ants are popular and mass-produced in South America (DeFoliart, 1999). In Mexico, edible insects are traditionally eaten in both rural and urban regions. However, increasing westernization of cities after Spanish conquest eventually has limited entomophagy largely to rural areas (Ramos‐Elorduy, 1997). Nevertheless, Mexican restaurants often served escamol, an insect dish fried with aromatic spices (DeFoliart, 1999). In Colombia, the Yukpa people prefer insects over meat, but it has been forced to decrease insect consumption owing to massive deforestation (Ruddle, 1973).
Sources: DeFoliart (1999), Ramos‐Elorduy (1997), Ruddle (1973).
The adoption of Western values among countries that traditionally consumed insects is correlated with a decrease in this behavior (DeFoliart, 1999; Ramos‐Elorduy, 1997; Yen, 2010). Therefore, Western attitudes toward edible insects must be adjusted to successfully expand the global market. Several explanations are available for why Western cultures generally did not develop entomophagy, including insect size, dispersed distribution, and seasonal unavailability (van Huis, 2016). Existing cultural differences were then used by Western cultures to denigrate traditional peoples, leading to an association of disgust with insect consumption (Looy et al., 2014). Furthermore, despite the fact that only 0.2% of insects are actually detrimental to human life, the persistent perceptions of harmful insects contribute to the reluctance in accepting entomophagy (van Huis, 2016). Further systemic efforts must be made to alter cultural attitudes through cooperation between academia, government, and industry.
Prospect of Insects as Food Resources
Despite the numerous advantages of insect consumption, the future of insect industry is unfavorable in Western societies (Sogari, 2015; van Huis, 2016), given that existing cultural distaste cannot be changed rapidly (DeFoliart, 1999). Fortunately, the increased consumer knowledge about edible insects also increases the willingness to pay for insect food (Piha et al., 2018). Continuous promotional efforts to increase exposure, coupled with development to enhance taste and appearance (Looy et al., 2014; van Huis et al., 2013), has successfully improved the negative perceptions in some Western countries. For example, consumers in Belgium increasingly accept insects as an excellent food source (van Thielen et al., 2019). Furthermore, the insect industry in the Netherlands has been successfully marketed, and the freeze-dried insect powder is sold as a meat replacement (Raheem et al., 2018). To increase acceptance, social, practical, and contextual factors affecting food consumption must be emphasized to consumers (House, 2016). Such efforts involve continuous education and promotion regarding the potential for edible insects to solve environmental, population, and land-availability problems today and in the future.
Here, we introduce available patents related to edible insects. The aim of this examination is to provide the objective information on the technological status of insect consumption, which will then be used for establishing strategic research and development. The patent applications related to edible insects have been filed yearly, with a notable uptick since mid-2010. Currently, 167 patents have been filed (Fig. 1). Thus, the research and development on entomophagy is being actively pursued. A sharp decrease in patent applications is observed starting from 2018, because it is probably that patents are released 18 months after application. In terms of the raw materials from edible insects, the most common patent applications are related to pupa, silkworm, brown goat, and grasshopper, in that order. Our analysis shows that most patents have been applying to protect food processing methods using edible insects.
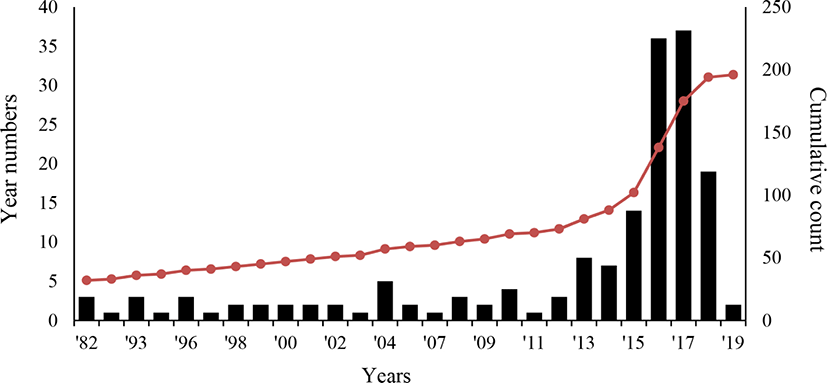
To better understand technological growth, we compared the number of applicants and applications across various time intervals (Fig. 2). We employed a time-series analysis based on patent number, using a technical life-cycle method (Little, 1981). The degree of competition is defined as applicant number in the analysis section. Production efficiency is a means of analyzing trends in time series by replacing application number or the extent of increase in applications.
In general, the technology life cycle consists of introduction, growth, maturity, and decay. Applicants and applications begin to appear during the introduction period. During the growth period, application numbers increase. During maturity, the number of applications received, and the number of continuing applications start to decrease. During decline, the number of applications remains steadily low. In our analysis of total effective patent applications filed, we divided the technical life cycle trends into four sections, approximately a four-year term: 1998–2002, 2003–2007, 2008–2012, and 2013–2018. We observed a continuous increase in applicant and application number from section one section to four. This pattern indicates that edible-insect-related technology may enter the stage of growth. Taken together, these analysis of patent trends suggest that the technologies related to edible insects are experiencing rapid and continuous growth.
Conclusions
Entomophagy could be the solution to an increasingly urgent food-security problem confronting the world. As we have described, numerous countries already use insects as alternative food protein and feed sources. Existing researches confirm the considerable nutritional and medicinal values of edible insects. Various strategies have been deployed to expand the edible-insect market and to counteract existing animus among Western societies toward entomophagy. As a result, patent numbers have increased continuously. In conclusion, this review shows the current status of edible insect remains insufficient to replace traditional animal foods worldwide, although edible insects have strong potential to become a primary source of nutrients.